In this post I share some of what I have learnt and worked out about how to reduce the carbon footprint of heating a house. There is an awful lot of misinformation and misleading advertising out there, and very few have reached the most sensible solution, particularly for fitting in older houses. I hope that this post will help you choose the best approach. I hope I have achieved the right balance between technical detail and simple explanation, and I would welcome feedback on that. Clarity may improve over time!
The biggest use of energy in housing is heating. The amount of heat required to maintain a temperature depends on the loss of heat from the building (e.g. heat loss through the walls). The energy required to provide that heat depends on the efficiency of the heat generator (e.g. boiler). The amount of carbon dioxide to heat the house depends on the CO2 released to produce the energy.
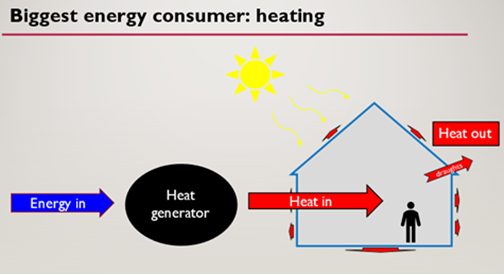
The majority of a house’s carbon footprint is through heating and so having the most efficient way of generating heat is essential. There are many different heat generation technologies. Some burn fuel and some use electricity to either directly converting it to heat (e.g. fan heater, storage heater) or by using the electricity to pump heat into the house from the environment.
Technical and economic comparison of different heat generation technologies
The following chart compares the heat generation efficiency of different heat generators, it shows how much energy is needed to provide 1kWh of heat. The best technology has the lowest value:
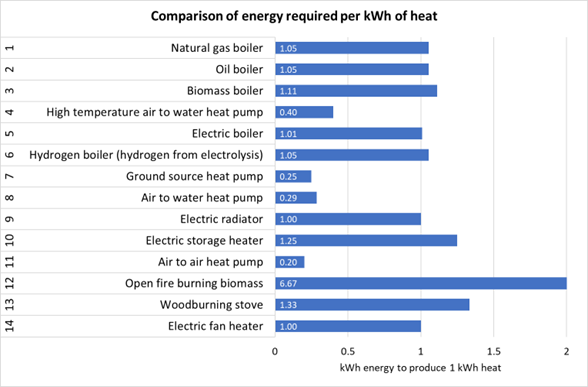
Most houses are currently heated via gas or oil-fired boilers. With that assumption, how easy are the different technologies to install?
1-6: can be considered as straight boiler replacements and offer the potential for relatively low disruption
7-8: can connect to an existing radiator system but would not provide the same heat input as the system they replace due to lower water temperatures. They will need additional measures such as increased insulation, or increasing radiator size. Installation will be disruptive, taking several days at least.
9-10: replace the present system and would need existing radiators to be removed to make space for the new heaters. Additional electrical wiring might be needed too. There would be some disruption.
11: can be installed quickly and with little disruption, perhaps in less than a day. Existing heating systems can be left in place.
12-14: are supplementary heating options which may be valuable to ‘top up’ in cold weather or are suitable for infrequently used areas.
From an efficiency point of view all of the heat pumps are very attractive, although from a disruption point of view the water systems are quite disruptive to install. The low disruption of fitting air to air heat pumps is very attractive.
I have compiled some typical installation costs of the different technologies:
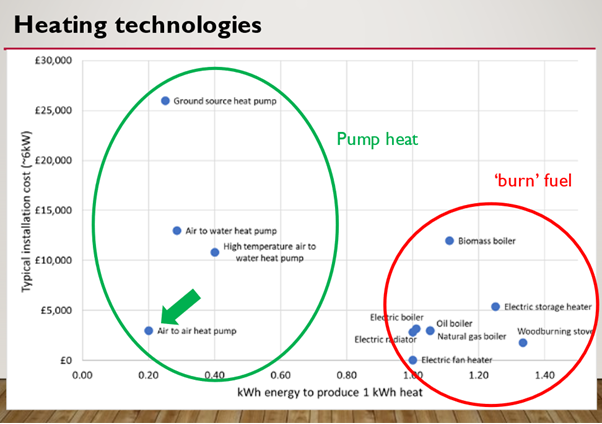
The water heat pump options are much more expensive than straight boiler replacement. However, air to air heat pumps offer not only the highest efficiency but at a comparable installation cost to traditional boiler replacement.
The government is currently offering £5000 off the cost and installation of an air source heat pump (ASHP systems must be hydronic (air-to-water) to be eligible under BUS. Air-to-air systems are not eligible.), £5000 off the cost and installation of a biomass boiler and £6000 off the cost and installation of a ground source heat pump, including water source heat pumps. This subsidy brings the costs closer, but they are still more costly and disruptive – perhaps explaining why uptake has been poor.
But what about running costs? The following chart shows the comparative running costs to provide 20MWh of heat, based on energy prices of:
Electricity 34.0p/kWh (government price cap value)
Gas 10.3p/kWh (government price cap value)
Oil 10.9p/kWh
Biomass 12.5p/kWh (price paid by a friend with a biomass boiler)
Hydrogen 56.7p/kWh (cost of electricity / 60% electrolysis efficiency)
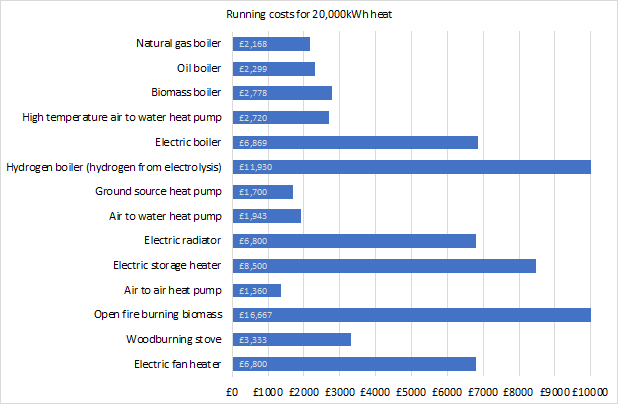
Although energy prices are rather unstable, this shows that the efficiency benefit of electrically driven heat pumps outweighs the price premium of the fuel. If electricity is generated ‘for free’ on site by solar panels perhaps, then the benefit will be larger.
Based on the data above, it is clear that heat pump technology must form the basis of the net zero heating approach, and that air-to-air heat pumps are by far the most attractive having the lowest installation and running costs and the highest efficiency. Additionally air to air heat pumps (air conditioning units) offer the benefit of being reversible and being able to cool the home in summer heat waves.
Heat pumps don’t work the same way as boilers
We need to understand the behaviour of heat pumps, because they don’t provide heat in the same way as burning fuel. Instead, they use a refrigerant cycle (just like on a fridge or freezer) to pump heat from a cold temperature to a hot temperature.
A heat pump has a certain amount of power. If you are pushing a car up a steep hill you can’t push such a heavy car as you could on the flat, and similarly a heat pump can’t pump so much heat when there is a big temperature rise between outside and inside. At cold outside temperatures, the heat pump capability reduces – they still pump heat, but just not quite so much.
However, as the outside temperature reduces the amount of heat leaking out of a building increases; at cold outside temperatures the amount of heat needed increases.
These two factors mean that the increase in design size of the heat pump for satisfactory heat in cold climates is rather bigger than for a conventional boiler. This is shown graphically here:
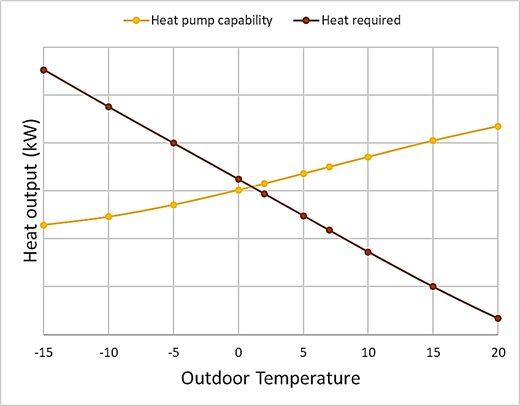
The following chart shows an example of how the heat pump rated output needs to increase as the design external temperature decreases, taking into account the need for more heat at lower temperature and the reduction in heat pump output at lower temperature.
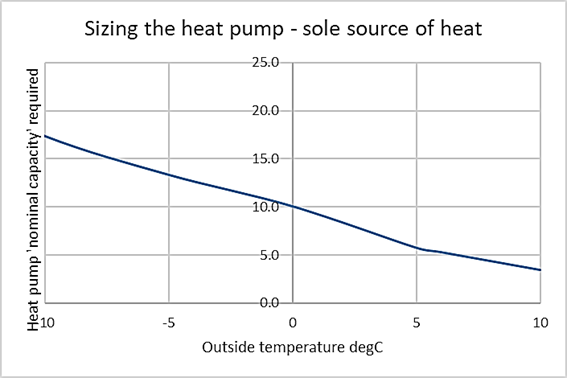
If we choose a heat pumps size that can cope with a very low outside temperature, then we need a big heat pump. So if we decide we must rely only on the heat pump at minus 10C then the heat pump will need to be 3.5 times bigger (and more expensive) than if we design the heat pump down to plus 5C.
If we were to design for 5C, then what do we do when the outside temperature drops to -15C? In simple terms we need to provide some additional supplementary heat. There are many ways of supplementing the heat from the heat pump, perhaps by using a wood-burning stove, or electric fans.
However, for the air to air heat pump solution, and attractive option in a ‘retrofit’ situation is to retain at least part of the current heating system as a ‘backup’ – for use only in the coldest weather. In other words: if you fit an air to air heat pump, don’t rip out the current central heating system – just avoid using it unless absolutely necessary.
This is not an option if the boiler is being replaced by an air to water system or a ground source heat pump system.
How many units, and where to put them?
In a traditional radiator based heating system, you can work out the heat needed for each room and put in a suitably sized radiator – each room having at least one radiator. Many radiators are only 1kW or less. If you are retrofitting air to air heat pumps you may want to economise significantly on that option, not least because the smallest internal unit is typically over 2kW.
In my own home, a traditional 3 bed semi, we have 8 rooms and had 8 radiators. We chose to fit a single internal unit in the hallway and our experience shows that we could heat all the rooms adequately simply by allowing the heat to dissipate through open doors into the occupied rooms. The temperature in the hallway needs to be higher than the temperature required in the other rooms – so if you follow a similar approach you will need to experiment with the ideal temperature setting on the air-con unit to sufficiently heat the other rooms. We also find that this approach does not heat the bedrooms to such a high temperature as the downstairs rooms. This suits us, but to avoid this, fit an internal unit on each level in the house.
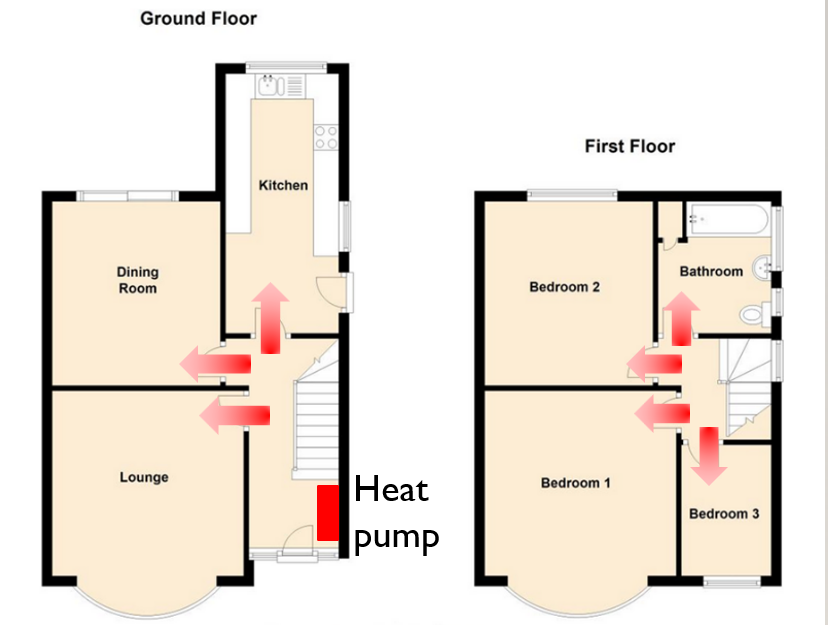
The picture below shows our internal and external units.
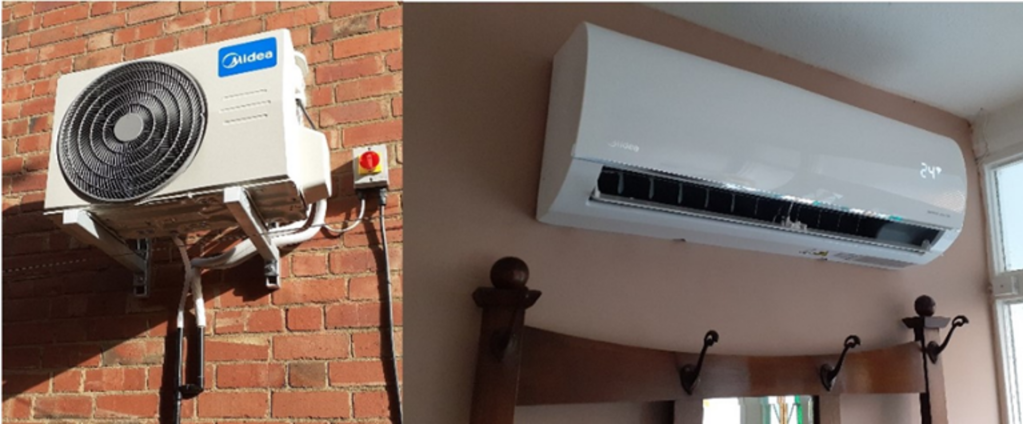
A single unit may not be sufficient for a larger our more complex house, but it is very likely that two or perhaps three internal units would be enough for most houses. There is perhaps as much art a science to where to put them, but think about where you would put a fan heater to best circulate the heat – that is in effect what these units are.
Note too that the internal unit does not need to be mounted on an external wall. Indeed, the most effective position may be in the middle of the house so that for the heat to finally escape it will pass through all the other rooms.
Often there is a single external unit for each internal unit, but a ‘multi-split’ system can provide many internal units run from a single external unit.
Our heat pump is size matches the steady state heat loss at around zero degrees outside temperature. In comparison, our boiler heat capability is perhaps five times as big. This high capacity is needed because traditionally the boiler would be turned off to save energy at night or when the house is unoccupied, and so a very high heat input would be needed to bring the temperature back up to comfort level. Since the heat pump is so efficient it can be run 24 hours a day, maintaining the temperature and avoiding the need for a short burst of high energy consumption. So, unlike a central heating boiler, the total heat pump capacity needs to be selected to match the steady heat loss from the house.
If you want an idea of how big a heat pump you might need, you could use an online radiator size calculator such as https://www.bestheating.com/btu-calculator for each room, and then add up the total for the house. This is likely to be rather higher than the steady state required, as the radiators need to be able to heat the house quickly from cold perhaps twice a day and to deal with the very coldest outside weather. I developed my own calculator to work out the heat loss, and taking into account the characteristics of a typical heat pump I can work out a heat pump size.
In conclusion – I hope that this is helpful, but it is a quite abbreviated summary of my experience and it may not be written as clearly as it should. If you have any comments or feedback, please get in touch. Thanks.